Contents of Article
- Summary
- Why is measuring force important in sports?
- What is force-velocity profiling?
- Why is force-velocity profiling important?
- How do you perform force-velocity profiling?
- Are there any issues with force-velocity profiling?
- Is future research needed with force-velocity profiling?
- Conclusions
- References
- About the Author
Summary
Force-velocity profiling is a simple and inexpensive way to assess an athlete’s force and velocity production capabilities during ballistic tasks such as jumping and sprinting. Through force-velocity profiling, a coach can identify whether an athlete is force- or velocity-deficient during a given movement (e.g. vertical jump), independent of their power capability. Based on the test results, targeted resistance training can then be implemented in order to reduce the athlete’s force or velocity deficiency, and improve their performance on that given task (e.g. vertical jump).
As a result, force-velocity profiling allows the strength and conditioning coach to tailor their athletes’ programmes more specifically by using detailed, objective information. Lastly, certain smartphone applications that require minimal set-up and provide instantaneous feedback can be used to assess the force-velocity profile of an athlete.
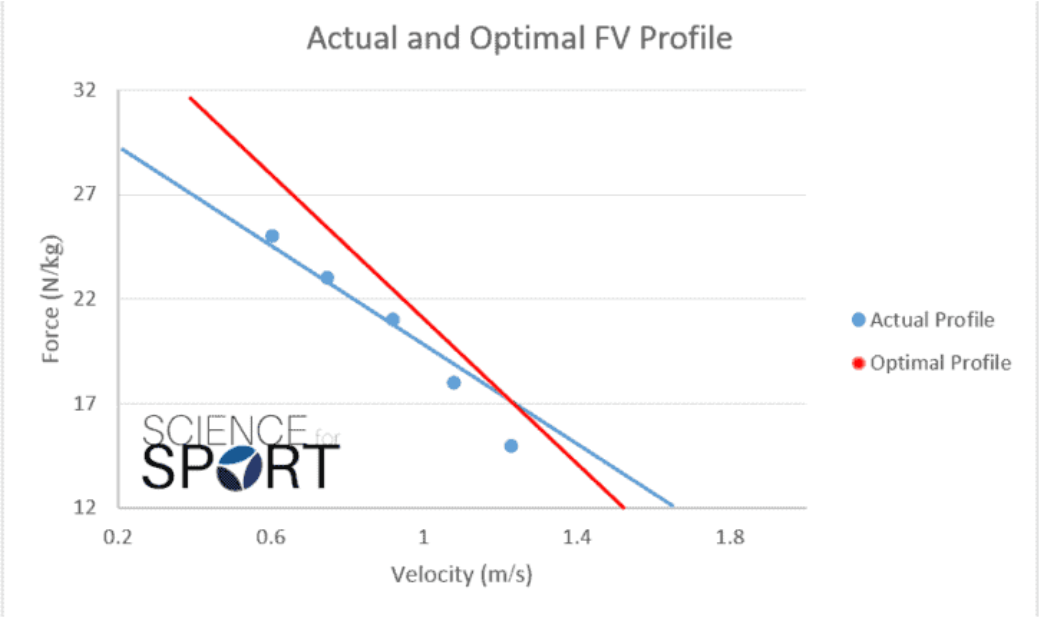
Why is measuring force important in sports?
The ability to produce high levels of muscular power is considered to be a vital component during many athletic and sporting activities such as jumping and sprinting (1, 2). Since power is the product of force multiplied by velocity (Power = Force x Velocity), it is therefore understood that these two components underpin the ability to be powerful.
Having said this, while two athletes may display similar power outputs, their force and velocity capacities may be markedly different. In theory, athletes are biased towards either strength (force) or speed (velocity), which may limit them in jumping (3) and/or sprinting movements (4). Therefore, measuring force and velocity – independent from power output – is useful for identifying whether an athlete is force- or velocity-deficient.
What is force-velocity profiling?
A force-velocity profile shows the relationship (curve) between the strength (force) and speed (velocity) of an athlete. Since power is a combination of force and velocity, the force-velocity profile also tells you something about athletic power (Power = Force * Velocity).
Force-velocity profiling (FVP) has gained popularity in recent years with both researchers and strength and conditioning coaches (4-10). Since the popularisation of FVP, multiple smartphone applications have been developed in order to identify an athlete’s force-power-velocity characteristics, and thus produce a theoretical FV profile. These applications are a simple way for coaches and researchers to measure an athlete’s force-power-velocity characteristics during a vertical jump or sprint.
This information can, therefore, inform coaches if an athlete is force- or velocity-deficient, as well as providing an optimal FV profile in which to work towards.
The principles of FVP are grounded by the Force-Velocity Curve, which if you are unfamiliar with, it is suggested that you read about the Force-Velocity Curve to better understand FVP.
As many coaches do not have access to expensive and complex laboratory equipment for which to test their athletes, FVP – via the use of smartphone applications – can provide a simple and inexpensive solution. FVP is often incorporated during performance testing sessions and can provide critical information to coaches if done periodically throughout a season.
Through profiling, coaches are provided with information regarding whether an athlete is force- or velocity-deficient in the given athletic task (e.g. jumping or sprinting). Furthermore, coaches are also provided with a theoretical and optimal FV profile, which can, therefore, allow them to implement training methods designed to target the deficiencies, and effectively optimise the athlete’s performance. This concept is better known as ‘targeted resistance training’.
FVP for Sprinting
The ability to accelerate, reach maximum velocity, and maintain it is critical during linear sprinting (5, 6). To understand FVP for sprinting, the horizontal force is an important concept to understand. In layman’s terms, and in regards to sprinting, the horizontal force is simply the force produced in the posterior direction as you sprint forward (5).
It has been reported the ability to produce horizontal force is a major factor during acceleration (8), as well as sprinting over longer distances (e.g. 100 m) (5, 6).
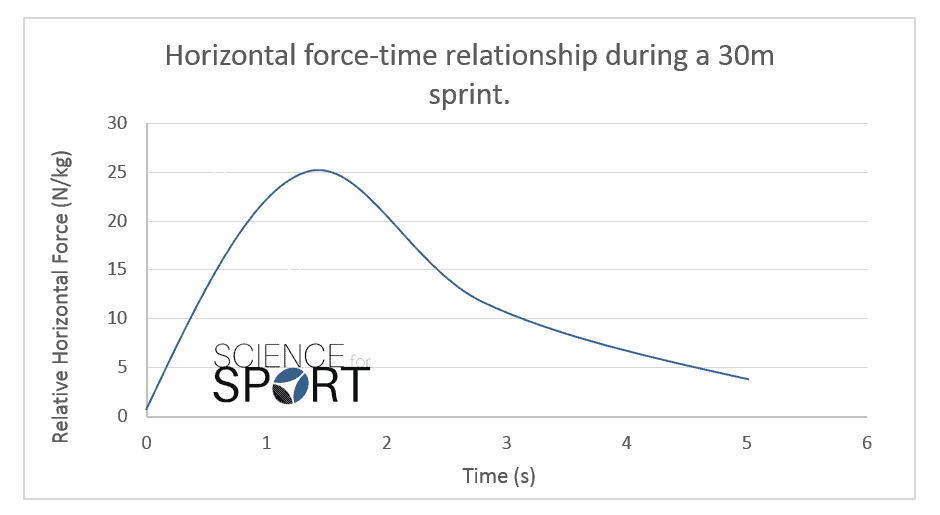
Although vertical force production peaks during maximal velocity, there is still a reliance on horizontal force production to move forward (6, 11, 13). Using FVP, a coach is able to calculate the ratio of force (RF) between vertical and horizontal ground reaction forces. During a sprint, this is calculated by dividing the horizontal force by the vertical force and measuring the slope at which the horizontal force decreases (5, 8).
Furthermore, the rate at which horizontal force declines is known as the rate of decrease in RF (DRF; Figure 2) (8). Whilst the loss in horizontal force production during sprinting cannot be entirely prevented, coaches can train the ability to sustain it and reduce the DRF (5).
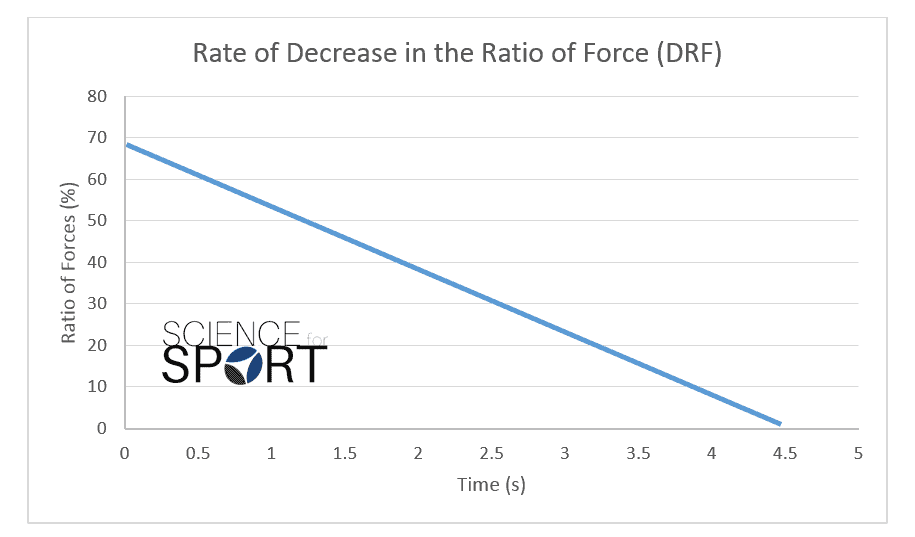
FVP for Jumping
Optimal vertical jump performance requires one to accelerate their body mass to reach the highest possible velocity in the shortest time frame available (9). Vertical jump height is also a valid reflection of an athlete’s lower-body maximal power output (14). As a result, an athlete’s maximal power output (i.e. jump height) can be improved by either increasing their ability to produce high levels of force (strength training) and/or by improving their movement velocity with low loads (ballistic training) (15-17).
Put simply, improving an athlete’s force and/or velocity capabilities will likely result in an increased jump height (i.e. power output). However, determining which component an athlete is deficient in (i.e. force or velocity), is where FVP becomes of use.
When creating an FVP for a vertical jump, information on the ‘actual’ and ‘optimal’ profiles are provided. The ‘actual’ profile reflects the current force and velocity capacities of the athlete, while the ‘optimal’ profile is what should be worked towards in order to increase the athlete’s jump performance. The optimal FV profile is calculated using a validated equation by Samozino et al. (2012) and colleagues which includes the maximal power output and vertical displacement of the centre of mass (9, 18, 19) (Figure 3).
The difference between the actual and optimal profile is known as the ‘FV imbalance’ (FVimb). This measure represents the magnitude and direction to which there is either a force or velocity bias. Decreasing the FVimb has been shown to increase vertical jump performance in both force-deficient and velocity-deficient athletes (3).
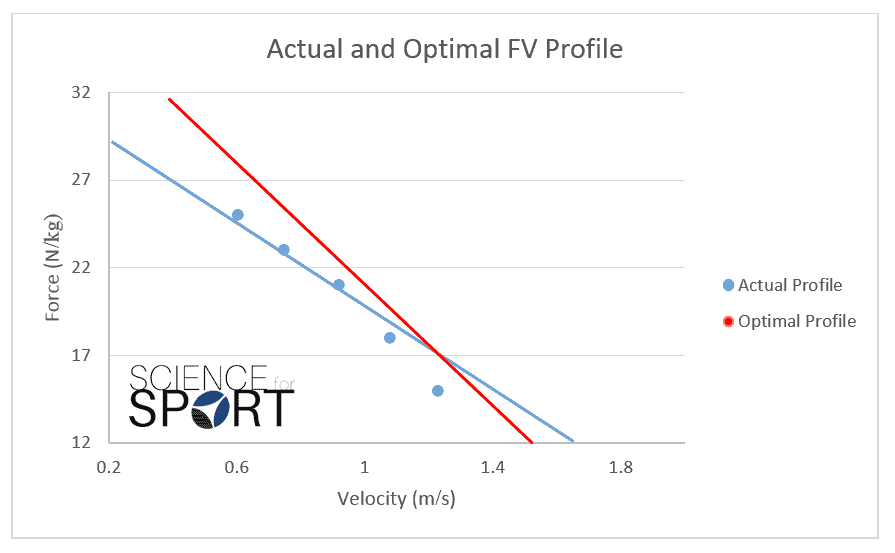
Why is force-velocity profiling important?
Targeted resistance training based on individual FV profiling has been shown to be an effective method for improving vertical jump performance (3). In a recent study, 84 subjects were profiled as either velocity-deficient, force-deficient, or well-balanced. From there, subjects were broken into sub-groups of force-focused training (if velocity deficient), velocity-focused training (if force deficient), a general training group (if well-balanced), and a control group.
All groups performed nine weeks of targeted resistance training specific to their deficiencies, with the goal of reducing any FVimb. Both the force-deficient and the velocity-deficient training groups significantly increased vertical jump performance, while the results of the general training group and control group were highly variable and unclear. The conclusions from this study suggest that FVP can be used by coaches to tailor their training programmes more specifically to their athletes’ needs.
Whilst this article primarily focuses on sprinting and jumping, other ballistic-type movements, such as the bench press throw (20), can also be subject to FVP. A recent study on rugby union players assessed the force-power-velocity characteristics between forwards and backs during the bench press throw (20). The results indicated the forwards were stronger and more powerful than the backs, and that the backs were biased towards velocity. This information can, therefore, allow the coach to tailor training programmes specifically to the athlete’s weakness.
Another interesting aspect of this study was that players were ranked in each position based on their performance in the bench press throws. At the discretion of the coach, informing the players of their rank may help rectify their bias towards either force or velocity and motivate them to work on their deficiencies. Using a ranking system, and periodically testing FV characteristics, may also be a way of monitoring players with objective data.
How do you perform force-velocity profiling?
FVP is a simple process that requires minimal equipment. The jumping and sprinting tests require different variables which are outlined below. Using reliable and validated smartphone applications (21, 22), a coach can quickly and easily calculate variables such as optimal force, optimal velocity, FVimb, RF, and DRF.
The detailed methods/instructions for calculating FVP in sprinting and jumping can be found in the following studies (4, 8, 10, 18):
- Samozino et al. (2015)
- Morin et al. (2016)
- Samozino et al. (2014)
- Samozino et al. (2008)
How to FV profile maximal sprinting
In order to create an FV profile for sprinting, the following items are needed: body mass (kg), height (m), and either distance-time data or speed-time data (minimum five splits for a given distance) which can be obtained with timing gates (4). It is also recommended testing should be performed indoors to ensure a consistent surface, and to prevent any impact of wind speed and/or temperature. In addition, visible vertical poles are also required in order to mark the distance for different splits (e.g. 5, 10, 15 m).
To assess a sprint FVP, the RF and DRF must be fully understood. RF is the percentage calculated by the amount of horizontal force produced divided by the vertical force produced throughout a sprint. A higher RF throughout the sprint is considered desirable as it suggests the athlete is applying higher amounts of horizontal force. However, as speed increases, the RF (%) will inevitably decline – typically referred to as the DRF. A lower DRF is considered desirable as this implies that the athlete is better at producing horizontal force as velocity increases.
How to FV profile maximal jumping
In order to create an FV profile for jumping, the following items are needed: body mass (kg), standing height (m), jump height (m), and two lower-limb length measurements: 1) at fully extended position (m); and 2) with knees bent at 90° (m). A minimum of five separate jumps with an additional load for each jump is required to create a profile. To choose loads, there must be an even distribution of additional load starting from 0 % body mass to the last load at which the participant can jump about 10 cm with (8). For example, the five loads you may choose are body weight (BW), 15 % BW, 30 % BW, 45 % BW, and 60 % BW.
To assess a jump FVP, the FVimb between the ‘actual’ and ‘optimal’ jump profile must be fully understood. The distance and direction between the actual and optimal FV slope are referred to as the FVimb (Figure 3). A greater FVimb (%) suggests the athlete is biased towards either force or velocity (i.e. force- or velocity-deficient), depending on the direction of the slope. A lower FVimb is considered desirable as it suggests the athlete is well-balanced in producing both force and velocity. When an athlete demonstrates a high FVimb, decreasing the number is often the priority and can result in an improved vertical jump performance (3).
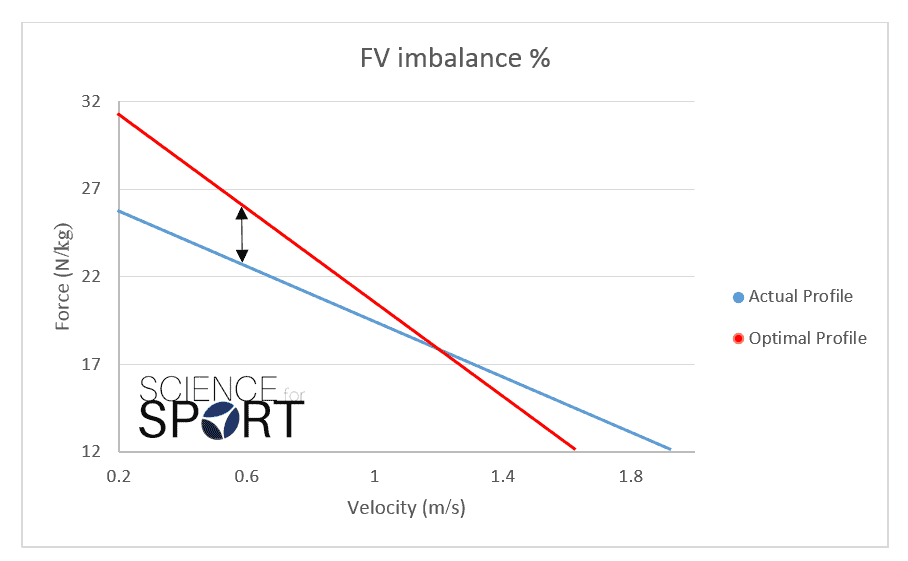
The equation used to calculate the FVimb (%) based on linear regression models of actual and optimal FVP is shown below (4, 9):
FVimb (%) = 100 (1 – FV actual / FV optimal)
The loaded jumps will provide a theoretical maximal force production value based on the loads used. Likewise, theoretical maximal velocity production will also be calculated based on the athlete’s time in the air during the jumps. With this information, the ‘actual’ FV slope can be calculated based on the loaded jumps, and from this, an ‘optimal’ FV slope can be calculated.
Are there any issues with force-velocity profiling?
Despite the promising concept and application of FVP, it does, however, still come with its faults. Some of these include:
- Linear regression: FVP uses linear regression to determine an optimal profile (23), however, it needs to be understood there is not a perfect correlation between force and velocity, despite linear regression assuming there is.
- Specificity: FVP is specific to the movement, and therefore, may not be specific to the sport. For example, a bilateral vertical jump is different from a lay-up jump in basketball. Optimal FVPs have been shown to be lower than actual FVP in world-class athletes (24). Chronic practice at a specific sport can create imbalanced FVPs, although this may not be limiting to performance (24) – take powerlifting, for example.
Is future research needed with force-velocity profiling?
Whilst there is emerging information and research supporting the efficacy of this testing and training method, it is very much in its infancy, and many more questions need to be answered. Some of which include:
- The effects of FVP on specific athletic populations.
- The effects of FVP on different ballistic-type movements (e.g. sled sprinting).
- How growth and maturation affect the FVP of young athletes around the period of peak height velocity.
- Whether FVP can be an effective tool to monitor performance and fatigue.
Conclusion
FVP is a useful tool for which strength and conditioning coaches can measure their athlete’s force and velocity capacities, independent of power. The optimal FV profiles generated can be used as training targets for the athletes in order to become more effective at jumping and sprinting.
It is important to understand the different variables associated with both jumping and sprinting. For example, with FVP in jumping, it is critical to decrease the FVimb in order to improve jump performance. Meanwhile, for sprinting, a lower DRF is desirable as it demonstrates the athlete is losing minimal amounts of horizontal force production as velocity increases.
FVP can be cost-effective, and can also be easily obtained using smartphone applications. However, like everything, FVP is not perfect and does have its limitations. It is not the be-all and end-all, but rather another tool that coaches can use to test athletes and perhaps programme more specifically. Having said this, coaches must decide if they feel there is enough value in this method in order to warrant its inclusion within the overall programme.
- Cronin, J., and Sleivert, G. (2005). Challenges in understanding the influence of maximal power training on improving athletic performance. Sport. Med. 35, 213–234. https://www.ncbi.nlm.nih.gov/pubmed/15730337
- Stone, MH., Moir G., Glaister, M., and Sanders, R. 2002. How much strength is necessary? Phys Ther Sport 3: 88–96. http://www.physicaltherapyinsport.com/article/S1466-853X(01)90102-6/fulltext
- Jiménez-Reyes P, Samozino P, Brughelli M and Morin JB. (2017) Effectiveness of an Individualized Training Based on Force-Velocity Profiling during Jumping. Front. Physiol. 7:677. https://www.ncbi.nlm.nih.gov/pmc/articles/PMC5220048/
- Samozino, P., Edouard, P., Sangnier, S., Brughelli, M., Gimenez, P., and Morin, J.B. (2014). Force-velocity profile: Imbalance determination and effect on lower limb ballistic performance. Int. J. Sports Med. 35,505–510. https://www.ncbi.nlm.nih.gov/pubmed/24227123
- Morin JB, Edouard P, Samozino P. (2011). Technical ability of force application as a determinant factor of sprint performance. Med Sci Sports Exerc. 43:1680-1688. https://www.ncbi.nlm.nih.gov/pubmed/21364480
- Morin JB, Bourdin M, Edouard P, Peyrot N, Samozino P, Lacour JR. (2012). Mechanical determinants of 100-m sprint running performance. Eur J Appl Physiol; 112:3921-3930. https://www.ncbi.nlm.nih.gov/pubmed/22422028
- Morin, J.B., Gimenez, P., Edouard, P., Arnal, P., Jiménez-Reyes, P., Samozino, P., Mendiguchia, J. (2015). Sprint Acceleration Mechanics: The Major Role of Hamstrings in Horizontal Force Production. Frontiers in Physiology, 6, 404. https://www.ncbi.nlm.nih.gov/pmc/articles/PMC4689850/
- Morin JB, Samozino P. (2016). Interpreting Power-Force-Velocity Profiles for Individualized and Specific Training. International Journal of Sports Physiology and Performance, 11 p 267-272. https://www.ncbi.nlm.nih.gov/pubmed/26694658
- Samozino, P., Rejc, E., DiPrampero, P.E., Belli, A., and Morin, J.B. (2012). Optimal force-velocity profile in ballistic movements–altius: citius or fortius? Med. Sci. Sports Exerc. 44,313–322. https://www.ncbi.nlm.nih.gov/pubmed/21775909
- Samozino P., Rabita G, Dorel S, Slawinski J, Peyrot N, Saez de Villarreal E, Morin JB. (2015). A simple method for measuring power, force, velocity properties, and mechanical effectiveness in sprint running. Scand J Med Sci Sports 6, 648-658. https://www.ncbi.nlm.nih.gov/pubmed/25996964
- Kugler F, and Janshen L. (2010). Body position determines propulsive forces in accelerated running. J Biomech 43: 343-348. https://www.ncbi.nlm.nih.gov/pubmed/19863962
- Weyand PG, Sternlight DB, Bellizzi MJ, and Wright S. (1985). Faster top running speeds are achieved with greater ground forces not more rapid leg movements. J Appl Physiol 89: 1991-1999, 2000. https://www.ncbi.nlm.nih.gov/pubmed/11053354
- Brughelli M, Cronin J, and Chaouachi A. (2011). Effects of running velocity on running kinetics and kinematics. J Strength Cond Res 25: 933-939. https://www.ncbi.nlm.nih.gov/pubmed/20703170
- Markovic, G., D. Dizdar, I. Jukic, and M. Cardinale. (2004). Reliability and factorial validity of squat and countermovement jump tests. J. Strength Cond. Res. 18(3):551–555. https://www.ncbi.nlm.nih.gov/pubmed/15320660
- Cormie, P., McGuigan, MR., Newton, RU. (2011). Developing maximal neuromuscular power: part 2—training considerations for improving maximal power production. Sports Med. 41(2):125. https://www.ncbi.nlm.nih.gov/pubmed/21244105
- Cronin, J., McNair, PJ., Marshall, RN. (2001). Velocity specificity, combination training and sport specific tasks. J Sci Med Sport. 4(2): 168-78. https://www.ncbi.nlm.nih.gov/pubmed/11548916
- McBride, JM., Triplett-McBride, T., Davie, A., Newton, RU. (2002) The effect of heavy- vs, light-load jump squats on the development of strength, power, and speed. J Strength Cond Res. 16(1):75-82. https://www.ncbi.nlm.nih.gov/pubmed/11834109
- Samozino, P., Morin, J.B., Hintzy, F., and Belli, A. (2008). A simple method for measuring force, velocity and power output during squat jump. J. Biomech. 41, 2940–2945. https://www.ncbi.nlm.nih.gov/pubmed/18789803
- Samozino, P., Rejc, E., Di Prampero, PE., Belli, A. and Morin, JB. (2014). Force–Velocity Properties’ Contribution to Bilateral Deficit during Ballistic Push-off. Med. Sci. Sports Exerc., Vol. 46, No. 1, pp. 107–114. https://www.ncbi.nlm.nih.gov/pubmed/24346189
- McMaster, DT., Gill, N., Cronin, J., and McGuigan, M. (2016) Force-velocity-power assessment in semi-professional rugby union players. J Strength Cond Res 30(4): 1118–1126. https://www.ncbi.nlm.nih.gov/pubmed/23838968
- Balsalobre-Fernandez, C., Glaister, M, Lockey, R. (2015). The validity and reliability of an iPhone app for measuring vertical jump performance. J Sport Science 33(15) pg. 1574-1579. https://www.ncbi.nlm.nih.gov/pubmed/25555023
- Romero-Franco, N. (2017). Sprint performance and mechanical outputs computed with an iPhone app: Comparison with existing reference methods. Eur J Sport Sci 17(4) pg. 386-392. https://www.ncbi.nlm.nih.gov/pubmed/27806673
- Cross, MR., Brughelli M., Samozino, P., Morin, JB. (2017). Methods of Power-Force-Velocity Profiling During Sprint Running: A Narrative Review. Sports Med 47 (7); 1255-1269. https://www.ncbi.nlm.nih.gov/pubmed/27896682
- Giroux, C., Rabita, G., Chollet D., Guilhem, G. (2016). Optimal Balance Between Force and Velocity Differs Among World-Class Athletes. Journal of Applied Biomechanics 32, 59-68. https://www.ncbi.nlm.nih.gov/pubmed/26398964